Mass photometry is transforming biomolecular analysis by allowing scientists to observe and understand the composition of single molecules in solution. Developed at the University of Oxford and commercialized by Refeyn, this technology uses laser-induced signals to determine the mass of biological compounds, including protein complexes, nucleic acids, and viruses, without labels or modifications. With real-time insights into biomolecular mass and interactions, mass photometry is emerging as a powerful tool for studying complex formations, binding events, and aggregation behavior.
Stephen Maughan, Product Manager at Refeyn, provides insights into the core principles of this technology, its unique applications, and its potential impact on biomolecular research.
How Mass Photometry Works
Maughan explains that mass photometry (MP) relies on interferometric scattering (iSCAT), a microscopy technique that visualizes nanoscale objects by analyzing laser light scattered by these particles. In Refeyn’s instrumentation, this involves shining a laser onto a microscopy coverslip, where samples of protein or other biomolecules or particles suspended in a buffer are introduced as droplets. “As the protein molecules land, they bind to the glass,” he says. “We record the scattered light from the protein, examining how that light interferes with the reflected light from the coverslip.”
The amount of light scattered by a molecule correlates with its polarizability, which, in proteins, depends on their mass because the refractive index and specific volume of each amino acid are sufficiently similar. As molecules in solution bind to a glass coverslip, incident light scatters, creating measurable patterns. These scattering events generate contrast signals, similar to ripples on a pond’s surface, which mass photometry captures. The contrast signals correlate directly with the molecular mass.
Bridging Gaps in Biomolecular Analysis
According to Maughan, this technique is particularly well-suited for protein analysis due to proteins' predictable interactions with laser light. “Proteins are all made up of amino acids, which respond in the same way to the laser illumination in terms of their refractive index. As a protein gets bigger, the contrast will increase linearly.”
In contrast to bulk measurement methods, mass photometry excels at analyzing individual particles, offering advantages over techniques such as size exclusion chromatography with multi-angle static light scattering (SEC-MALS) or dynamic light scattering (DLS). “You’re measuring the mass instead of the size, which differentiates the technique from DLS or nanoparticle tracking analysis (NTA),” explains Maughan. “Because it's single-particle, you're very sensitive to low abundance populations within your sample.”
The simplicity and speed of mass photometry make it accessible and efficient. “The user interface is just a coverslip with a little well,” Maughan says. “You pipette in 20µL sample and press go; in just over a minute, you have your data.” Most users become proficient within half a day, he adds. “The data analysis is very intuitive as there are no charge components like in mass spec or Native PAGE—it’s just the mass distribution of your sample.”
An additional advantage of mass photometry is its ability to perform measurements under native conditions. “Unlike an SDS-PAGE gel where you’re separating out all the different components of your complex, mass photometry keeps that complex together,” points out Maughan. “So you're measuring everything as it is in the buffer.”
Unique Applications in Life Sciences
In protein analysis, mass photometry offers advantages that are challenging to achieve with other methods. Maughan notes that one area gaining traction is bi- and multispecific antibodies. Researchers aim to identify stoichiometries under different conditions, such as varying antigen-to-antibody ratios.
“Because we measure mass with good resolution, we’re able to differentiate between what’s bound to each arm of the antibody,” says Maughan. “You can see the unbound antibody, one molecule bound, and then both molecules bound—something that is difficult to achieve with methods such as surface plasmon resonance (SPR).”
Another significant application is in the analysis of adeno-associated viruses (AAVs). “We focus on distinguishing between empty, full, and partially filled AAV populations,” Maughan explains. “This works because we measure mass, not size—the viral capsid’s size remains constant, but its mass changes with the payload.” Mass photometry sets itself apart from SEC-MALS by detecting populations of partially filled AAVs, a capability typically only possible with complex methods such as mass spectrometry or analytical ultracentrifugation (AUC), which are more challenging and costly to use.
The Evolution of Mass Photometry
While this technology has demonstrated utility in various applications, ongoing work aims to further expand its capabilities. Maughan notes that recent developments are making mass photometry even easier and more efficient to use for specific research needs, such as measuring antibody aggregation. “We just introduced a new software module where rather than results appearing as a mass histogram (which is what a typical mass photometry method will yield), you instead see the ratios between your monomer, dimer, trimer, and larger populations,” he explains.
A priority for Refeyn is to broaden the use of mass photometry in GMP-compliant environments and ensure compliance with 21 CFR Part 11 (a regulation for secure electronic records and signatures). “This capability would allow the technology to be used not just in the development of new therapies but also in their manufacture,” asserts Maughan. Having already introduced software that enables AAV empty/full analysis in GMP-compliant environments, the company is looking to bring this capability to other applications in the future. It is also looking at enhancing system throughput, improving automation, and refining specifications such as limit of detection (LOD) and resolution.
Adopting Mass Photometry Methods
For researchers considering integrating mass photometry into their workflows, it’s essential to evaluate a few key factors to ensure successful adoption. Maughan advises that a primary consideration is the sample’s mass range—mass photometry works best for larger proteins and complexes, typically from 30–50 kilodaltons up to 5–6 megadaltons.
Another important consideration is sample concentration. Because the technique operates in the nanomolar range, you’ll often need to dilute your samples more than you might with other methods, which can impact complexes with low affinities. That said, there is a product available that enables rapid dilution while maintaining measurement accuracy for low-affinity complexes.
Additionally, it’s essential to be mindful of your buffers and sample cleanliness, as the sensitivity of mass photometry can reveal unexpected particulates that may interfere with results. Using clean buffers is crucial to avoid unwanted artifacts.
Maughan also notes that mass photometry offers some key advantages when used as an orthogonal technique, for example, in place of AUC alongside SEC-MALS. Because mass can provide valuable information in many contexts, mass photometry can be used in many ways. It's particularly suitable when there is a need for rapid, cost-effective assessments without the effort of labeling or other sample preparation.
Looking Ahead
By allowing researchers to study biomolecules in their native state without modification, mass photometry provides a powerful tool for understanding molecular composition, aggregation, and binding. This unique capability continues to pave the way for breakthroughs across fields such as structural biology, drug development, and beyond.
Meet the Expert
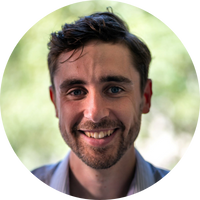
Stephen Maughan
Stephen is a Product Manager with Refeyn, a pioneer of mass photometry, a disruptive technique for quantifying the mass of biomolecules. He has 13 years of experience in instrument development for biophysical characterization across industry and academia, working on techniques such as dynamic light scattering, resonant mass measurement, and super-resolution microscopy. Stephen has a degree in Systems Engineering from Loughborough University and recently completed his PhD in Biochemistry at Oxford University.