In April 2024, the Environmental Protection Agency (EPA) announced a landmark ruling that requires municipal water systems across the United States to reduce the presence of six common perfluoroalkyl and polyfluoroalkyl substances (PFAS) in drinking water to near undetectable levels. Public water systems now have five years to meet the EPA’s new limits on these toxic compounds, commonly referred to as ‘forever chemicals’ due to their persistence in the environment and the human body.
The finalized drinking water standards impose strict limits on the two most prevalent forms of PFAS: perfluorooctanesulfonic acid (PFOS) and perfluorooctanoic acid (PFOA), setting them at four parts per trillion (ppt)—a significant reduction from the previous 70 ppt. Other types of PFAS are capped at 10 ppt.
In a discussion with Separation Science, Craig Butt (Senior Manager, Applied Markets, Global Technical Marketing at SCIEX) highlights that while labs possess the expertise to comply with the new EPA PFAS regulations, substantial problems persist. These include how to minimize contamination risks during PFAS measurements, identifying the myriad PFAS compounds not regulated by the EPA, and dispelling widespread misinformation about these chemicals.
The Realities of Part-Per-Trillion Detection
The U.S.’s Unregulated Contaminant Monitoring Rule (UCMR) program, part of the Safe Drinking Water Act, began to include PFAS, specifically under UCMR3, in 2013. This initiated a decade-long development of analytical testing methods, reflecting a mature understanding and capability within labs across the nation. Additionally, the evolution of EPA methods from 537 to 537.1, and subsequently to 533, significantly advanced standardization of testing protocols.
“While these methods have their challenges, they represent well-developed, regulated approaches for testing PFAS. Laboratories—from contracted to governmental and even smaller municipal facilities—have been employing these standardized methods for quite some time,” notes Butt. “It’s important to recognize that we’re not starting from ground zero with these regulations.”
For standard detection of PFAS in drinking water, Butt recommends liquid chromatography with a mid-range mass spectrometer, such as the SCIEX 5500+ system. “You don’t need a high-end system if you’re going to follow the EPA drinking water methods, because they already incorporate rigorous sample preparation steps. A mid-range triple quad is more than adequate to hit low parts per trillion sensitivity.”
However, Butt cautions that achieving part-per-trillion detection necessitates vigilance regarding contamination risks. Routine lab hygiene, dust, and common consumables can introduce background PFAS signals, complicating test results. He emphasizes a simple rule—the more a sample is manipulated, the greater the contamination risk. Consequently,
standard sample preparation steps, such as nitrogen blowdown to concentrate samples, may need re-evaluation.
Alternative Methods for PFAS Detection
To address these challenges, Butt played a pivotal role in a SCIEX technical study that explored alternative PFAS quantification methods in water using LC-MS/MS. The first method, aligned with EPA Method 537, utilized weak-anion exchange solid-phase extraction (SPE) to concentrate samples for HPLC separation. In contrast, the second method involved diluting samples in methanol and performing a large-volume direct injection of 950 μL on wider diameter columns.
Both techniques quantified PFAS at levels between 1–10 ng/L across 17 compounds. While the SPE method offers compatibility with stringent EPA requirements through enhanced sample concentration, the direct injection method simplifies the process and minimizes the risk of contamination, making it efficient for routine testing.
“EPA methods prescribe very specific procedures,” explains Butt. “In contrast, European standards are performance-based, offering flexibility as long as the results maintain high data quality. Using large volume injections takes advantage of the instrument’s sensitivity, streamlining the process and reducing potential headaches.”
For labs considering direct-injection processes, the SCIEX 7500 system offers advantages. Recent comparisons reveal that the SCIEX 7500 system enhances PFAS analysis with its precise detection of lower-level compounds and isomers. Its superior sensitivity provides higher signal-to-noise ratios, enabling quantification at trace levels. The system also simplifies sample preparation and reduces sample use, and the intuitive SCIEX OS software
streamlines data acquisition and processing.
Additionally, the SCIEX 7500 system facilitates sensitive analysis from low-volume samples, achieving ng/L detection limits using only 100 μL of sample volume. This capability empowers labs to adapt quickly to different sample sizes and concentration levels.
Advancing Non-Targeted PFAS Analysis
While routine EPA drinking water methods are manageable for skilled analysts, identifying unlisted, unknown PFAS compounds presents a different approach. Butt highlights the necessity for innovative hardware and sophisticated algorithms capable of processing vast amounts of MS data to detect PFAS variants, estimated to be around 10,000 in number.
For non-targeted analysis, the preferred choice is the SCIEX QTOF, which merges triple quadrupole technology with the high-resolution and accurate mass capabilities of time-of-flight mass analyzers. These systems employ SCIEX’s SWATH data-independent acquisition technology, enabling comprehensive, high-resolution MS/MS data collection across complex matrices.
Instruments such as the X500 QTOF system and the ZenoTOF 7600 system are particularly suited for this work. The latter enhances the sensitivity of fragment ion spectra through its Zeno trap technology, making it ideal for the complex task of identifying unknown PFAS compounds.
Tracing PFAS Sources
A key area of interest is how non-targeted analysis might intersect with new EPA regulations on PFAS. According to Butt, one way is to act as a chemical detective, identifying sources of contamination through unique signatures. For instance, he notes a case where the EPA used this method to pinpoint a New Jersey chemical manufacturer responsible for PFAS contamination, identifying them by distinct MS/MS fragmentation patterns.
“When a drinking water supply falls out of compliance, there’s inevitably a search for the root cause—whether it’s landfill leaching, a wastewater treatment plant, or firefighting foams from the local airport,” says Butt. “From a liability standpoint, someone’s got to pay for it.”
With a multidisciplinary research background focused on how PFAS compounds enter wildlife and travel to the Canadian Arctic, Butt takes a frontline role in communicating the PFAS problem to scientists and nonspecialists alike.
“Pesticides are straightforward for most people to understand: they are applied to fruits and vegetables, and you’re exposed. However, PFAS behaves differently,” explains Butt. “The PFAS detected in our blood and Arctic wildlife are not the same types used in commercial products. Instead, they are degradation products that form when PFAS break down and transform, making them more challenging to track. They contaminate the water supply and household dust and can volatilize from objects such as couches, undergoing atmospheric changes and dispersing globally.”
“Making that connection, the journey from the factory to the consumer, is a significant hurdle to overcome in people’s minds,” he adds.
Adam Dickie is a science writer for Separation Science. He can be reached at adickie@sepscience.com.
Craig Butt
Senior Manager, Applied Markets, SCIEX
Craig obtained his PhD in environmental chemistry at the University of Toronto, under the co-supervision of Dr. Scott Mabury and Dr. Derek Muir (Environment Canada), where he investigated the fate of PFAS in biological systems. Craig was then an NSERC postdoctoral research fellow and research scientist at Duke University, under the guidance of Dr. Heather Stapleton. Among other interesting topics, Craig's postdoctoral research involved measuring human PFAS exposure in serum, hand wipes, and wristbands. In his 20 years of mass spectrometry experience, Craig has published 48 peer-reviewed manuscripts, many of which are on PFAS, are globally recognized, and are highly cited. Within SCIEX, Craig is the applications lead for all things PFAS and has contributed to a variety of PFAS-specific applications, including EPA Methods 537.1 and 533.
Sponsor:
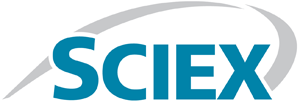
This article is featured in our June 2024 publication, Innovation and Sustainability in Modern Analysis. Find out about the latest innovations and sustainable advances in mass spectrometry, chromatography, and related techniques.