MALDI Imaging is established as a core technique in many areas of clinical research. It allows deep exploration of metabolic activity, unlocking the biological mechanisms that regulate cellular interactions, maintain tissue homeostasis, and influence the progression and treatment of disease. Now, new tools are enabling researchers to delve even deeper into the analysis and visualization of molecular distributions within thin tissue sections. These span a spectrum of molecules, from metabolites, lipids, glycans, and peptides, with no need for molecular markers or labeling, to larger proteins with appropriate labeling strategies. These latest advances give scientists a powerful tool that completes a multiplexed, multimodal, and multiomic analysis of a whole slide in under one hour.
Introduction
Matrix-assisted laser desorption ionization (MALDI) Imaging is uniquely suited to advance clinical research by measuring and visualizing the spatial distribution of endogenous and exogenous molecules within their native tissue context. The idea of measuring the spatial distribution of molecules on tissue sections is not new.[1] Immunohistochemistry (IHC) and in situ hybridization (ISH) have been in use for more than 50 years, but limitations of these methodologies—specifically, difficulties using large numbers of dyes or labels—hinder any ability to scale up to multiplexed analysis. As a result, since work in the early 1990s established the principle and initial applications of MALDI Imaging,[2] and the breakthrough in 2005 that enabled the accurate co-location of MALDI images with other non-mass spectrometry imaging data, MALDI Imaging has been considered a vital tool in the armory of clinical research.[3]
The technique has been further developed and improved over the last two decades, and for many researchers working in areas such as cell biology, oncology, or neurodegenerative diseases, the molecular maps generated by the current generation of MALDI Imaging instruments can provide valuable insights into various aspects of basic and translational biology, including spatial tumor and tumor microenvironment biology, pharmacological interventions, and patient stratification.
MALDI Imaging enables:
- Untargeted, label-free analysis of metabolites, lipids, glycans, and peptides
- Targeted analysis of larger protein molecules using appropriate labels
The technique is designed to chart the spatial distribution of molecules within a thin sample— most often a fixed fresh-frozen or formalin-fixed paraffin-embedded (FFPE) tissue section. A state-of-the-art MALDI Imaging dataset comprises a huge number of spectra (usually 5,000–50,000 spectra), with each raw spectrum representing intensities measured at a large number (usually 10,000–100,000) of positions and describing up to hundreds of different molecules.[4]
The focus of recent advances in both instrumentation and bioinformatics approaches has enabled improved performance, including the ability to achieve single cell spatial resolution,[5] 3D-tissue image reconstruction,[6] and the precise identification of different isomeric and isobaric molecules.[7] Arguably, the most significant development in MALDI Imaging in recent years has been its close integration with single cell omics as discussed in this recent review.[8]
What’s next in MALDI Imaging?
[AC1] Having attended many of the dedicated ‘future impact of spatial biology’ conferences in recent years, and spending time with industry experts in the field, there has been a clear consensus and a wish list regarding the need for future innovation:
- More plex and further increase in resolution without any loss of sensitivity
- Multiomics in one run
- More sophisticated bioinformatics
Against this background, a high performance MALDI IHC workflow has recently emerged, offering an integrated approach to multiplexed, multimodal, and multiomic spatial imaging.[9] Specifically, this integrated technology provides a platform to image 100+ protein biomarkers on a whole slide in around 40 minutes, at a resolution of 5 µm. Fluorescence microscopy, histopathological staining, and MALDI Imaging are integrated on one tissue section with no conjugation or metal tags required. IHC staining uses standard protocols and methods.[10]
How MALDI IHC works
MALDI IHC involves the use of novel photocleavable mass-tags linked to antibody probes, facilitating the development of high-throughput, multimodal, and multiomic MALDI workflows capable of simultaneously visualizing both small molecules and intact proteins within the same tissue specimen.
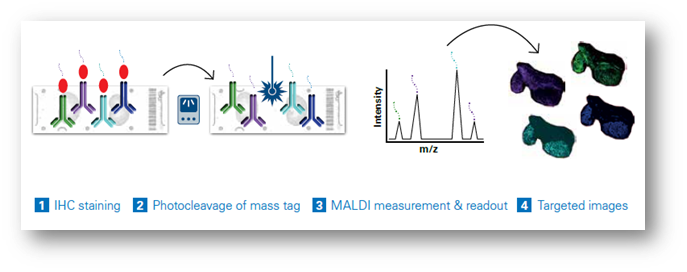
Dual-labeled antibody probes enable the combination of multimodal mass spectrometry (MS) and fluorescent imaging for precise examination of specific intact proteins. The latest integrated workflows permit the simultaneous analysis of MALDI Imaging with IHC (Figure 1), permitting the simultaneous analysis of more than a hundred distinct biomarkers in a single scan, eliminating the need for repetitive cycling.
Each probe carries a unique mass, facilitating the generation of highly multiplexed images. These images can be seamlessly integrated with other imaging techniques for multimodal data analysis or subsequent MALDI Imaging sessions, offering a comprehensive perspective on small molecules, lipids, glycans, intact proteins, and other elements within the same tissue sample (Figure 2). This capability permits the spatial mapping of metabolic pathways associated with specific proteins of interest and the quantitative evaluation of protein activity. As a result, researchers can precisely co-localize small-molecule drugs and their corresponding targets, including associated biomolecules involved in the cellular response to these drugs. Studies using MALDI IHC workflows have shown the ability to provide high-plex, multiomic, and multimodal imaging of tissues at a spatial resolution as low as 5 µm.[11] (Figure 3)
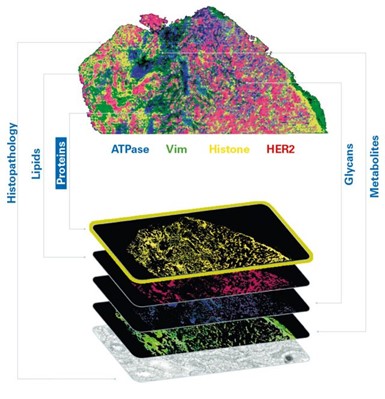
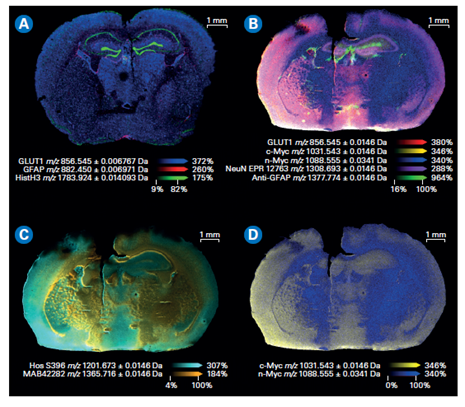
Figure 3. Composite color plots of extracted ion images of the mass tags used in a MALDI IHC workflow. A) Multiomics demonstration with lipids and drug target being imaged with a MALDI IHC workflow.[AC2] B) Composite color plots of extracted ion images of additional multiplexed antibody tags from the MALDI IHC workflow. C) and D) Composite ion images using select mass tags to specifically compare targeted proteins of interest for this system.
Advanced applications of MALDI IHC
MALDI IHC shows promise for use in the fields of disease-based research, therapeutics, and precision medicine. Concurrently examining proteins and small molecules within the same tissue can assist in uncovering new biomarkers linked to diseases, offering significant potential for early detection, prognosis, and tailored medical interventions. Researchers have demonstrated up to 121-plex MALDI-IHC “on mouse brain, human tonsil, and breast cancer tissues specimens, reflecting the known molecular composition, anatomy, and pathology of the targeted biomarkers.” [12] The same study has shown that novel, dual-labeled, fluorescent, photocleavable mass-tags (PC-MTs) antibodies and label-free small-molecule MALDI Imaging greatly extend the capability of this approach.
Additionally, these latest integrated imaging technologies offer the ability to delve into the intricate relationships between cancer cells and their microenvironment and can serve as powerful tools for unraveling the multifaceted dynamics at play in the tumor microenvironment. By examining the role of proteins and small molecules in processes such as tumor growth, invasion, and response to therapy, MALDI IHC can contribute significantly to the understanding of cancer biology. Researchers can identify potential biomarkers that indicate disease progression and tailor therapies to individual patients, providing insights into the development of more targeted and effective cancer treatments.
In neurobiology, MALDI IHC offers a window into the distribution of neurotransmitters, neuropeptides, and associated proteins within the intricate landscape of the brain. This capability is proving its worth in the study of neurological diseases and functions by uncovering the molecular underpinnings of conditions such as Alzheimer's disease, Parkinson's disease, and various psychiatric disorders. By mapping the distribution of these molecules, researchers can better grasp the complex mechanisms that drive both healthy brain function and pathological processes. This knowledge opens new avenues for the development of therapies and interventions aimed at addressing neurological disorders.
MALDI IHC also can be used to investigate the distribution and localization of drugs, metabolites, and their effects on proteins within tissues. Gaining a comprehensive understanding of how toxic compounds are distributed in the body and their consequent impact on tissues is of paramount importance in the field of toxicology. This comprehensive assessment can uncover mechanisms of toxicity, identify potential biomarkers of harm, and enhance the ability to predict and mitigate the adverse effects of harmful substances. In pharmacokinetics, MALDI IHC can provide insights into how drugs are metabolized, absorbed, and distributed in the body, shedding light on their efficacy and uncovering potential side effects. Furthermore, in drug development studies, the technique enables researchers to investigate how new compounds interact with tissues, offering a means to evaluate their safety and efficacy.
Looking ahead
In the last 25 years, MALDI Imaging has developed from a novel approach with clear potential to a routine technique for clinical research. The technology is being developed to offer a high performance, integrated solution that delivers intuitive workflows and high quality data, growing our understanding of complex biological processes that can ultimately help improve our quality of life.
References
[1] Eisenstein, M. (2022). Seven technologies to watch in 2022. Nature, 601, 658–661.
[2] Caprioli, R. M., Farmer, J. B., & Gile, J. (1997, December 1). Molecular imaging of biological samples: localization of peptides and proteins using MALDI-TOF MS. Analytical Chemistry, 69(23), 4751-4760. https://doi.org/10.1021/ac970888i
[3] Bruker. MALDI Imaging - Applications in Academia & Life Science. Retrieved from https://www.bruker.com/en/applications/academia-life-science/imaging/maldi-imaging.html
[4] Alexandrov, T. "MALDI imaging mass spectrometry: statistical data analysis and current computational challenges." BMC Bioinformatics 13, suppl. 16 (2012): S11. https://doi.org/10.1186/1471-2105-13-S16-S11
[5] Zhu, X., Xu, T., Peng, C., & Wu, S. (2022, February 3). Advances in MALDI Mass Spectrometry Imaging Single Cell and Tissues. Frontiers in Chemistry, 9, 782432. https://doi.org/10.3389/fchem.2021.782432
[6] Andersson, M., Groseclose, M. R., Deutch, A. Y., & Caprioli, R. M. (2008, January). Imaging mass spectrometry of proteins and peptides: 3D volume reconstruction. Nature Methods, 5(1), 101-108. https://doi.org/10.1038/nmeth1145
[7] Soltwisch, J., Heijs, B., Koch, A., Vens-Cappell, S., Hohndorf, J., & Dreisewerd, K. (2020). MALDI-2 on a trapped ion mobility quadrupole time-of-flight instrument for rapid mass spectrometry imaging and ion mobility separation of complex lipid profiles. Analytical Chemistry, 92(13), 8697–8703. https://doi.org/10.1021/acs.analchem.0c01747
[8] Zhang, H., Delafield, D. G., & Li, L. (2023). Mass spectrometry imaging: The rise of spatially resolved single-cell omics. Nature Methods, 20, 327–330. https://doi.org/10.1038/s41592-023-01774-6
[9] Analytical and Bioanalytical Chemistry (2023) 415:7011–7024. https://doi.org/10.1007/s00216-023-04983-2
[10] Frontiers in Chemistry. (2023, May 2). Analytical Chemistry Section, Volume 11, Article 1182404. https://doi.org/10.3389/fchem.2023.1182404
[11] Lim, M. J., Yagnik, G., Henkel, C., Frost, S. F., Bien, T., & Rothschild, K. J. (2023). MALDI HiPLEX-IHC: Multiomic and multimodal imaging of targeted intact proteins in tissues. Frontiers in Chemistry, 11, 1182404. https://doi.org/10.3389/fchem.2023.1182404
[12] Yagnik, G., Liu, Z., Rothschild, K. J., & Lim, M. J. (2021). Highly Multiplexed Immunohistochemical MALDI-MS Imaging of Biomarkers in Tissues. Journal of the American Society for Mass Spectrometry, 32(4), 977–988. https://doi.org/10.1021/jasms.0c00473